Research and development of synthetic biomolecules: an innovative path to whole cell and enzymatic synthesis
Synthetic biology: A new chapter in molecular manufacturing
In today’s era of rapid development of science and technology, synthetic biology is like a rising star, is reshaping the pattern of molecular research and development with its unique charm.
As a cutting-edge science that brings together the wisdom of biology, chemistry, engineering and other disciplines, synthetic biology is committed to carefully constructing new biological systems or optimizing existing biological systems from the most basic elements, using the concepts and methods of engineering.
In the field of molecular research and development, synthetic biology has shown unparalleled potential, opening the door to a new world.
It allows scientists to hold a magical “molecular paintbrush” that can precisely design and synthesize molecules with a variety of unique functions according to specific needs, whether it is an innovative drug for solving difficult diseases in the field of medicine, a new material with excellent performance in the field of materials science, or a clean and efficient bioenergy in the field of energy.
Synthetic biology has had an impressive performance.
In this grand scientific and technological blueprint, whole cell synthesis and enzymatic synthesis are undoubtedly the two most dazzling pearls, with their own unique advantages, become the core driving force to promote the vigorous development of synthetic biology, leading us to continue to explore the infinite possibilities of molecular manufacturing.
Whole Cell Synthesis: Building Mini “factories”
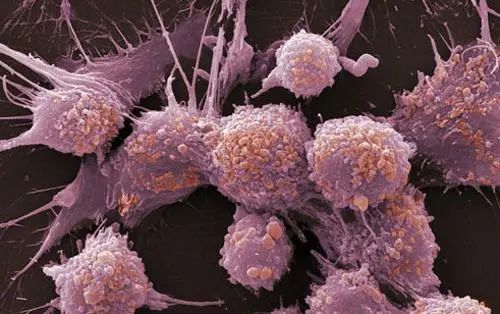
Whole-cell synthesis is like carefully building a sophisticated “molecular factory” in the microscopic world.
In this fascinating field, scientists use living cells as natural “production workshops,” cleverly harnessing the complex mechanisms inherent inside cells and transforming them into powerful engines for efficient synthesis of target molecules.
Take E. coli, a common microbe that is a star performer in synthetic biology.
Scientists have used gene editing technology to precisely modify the genes of E. coli, turning it into a “professional factory” for producing insulin.
Originally, Escherichia coli did not have the ability to secrete insulin, but after clever design, the human insulin gene was successfully introduced into the genome of Escherichia coli.
E. coli is like an intelligent robot that receives precise instructions, transcribs and translates insulin precursors methodically according to the set program, and then goes through a series of elaborate subsequent processing steps to finally produce medical insulin that is no different from the insulin secreted by the body itself.
This breakthrough not only greatly reduces the production cost of insulin, so that more patients with diabetes can use the affordable lifesaving drugs, but also vividly interprets the great potential and broad prospects of whole cell synthesis technology in the field of medicine.
Look at the biosynthesis of artemisinin, which is also a classic work of whole cell synthesis.
Artemisinin, as a special drug to treat malaria, used to be mainly extracted from natural artemisinin plants, but this method is restricted by many factors such as long plant growth cycle and easy to be affected by the environment.
To overcome this problem, scientists have taken a different approach, looking at microbial cells.
They dug deep into the pathway of artemisinin biosynthesis, accurately identified key enzyme genes involved, and cleverly introduced these genes into the cells of microorganisms such as yeast or E. coli.
These genetically modified microbial cells, as if endowed with magic, can use simple carbon and nitrogen sources under suitable culture conditions to continuously synthesize artemisinin and its precursors.
In this way, the production cycle of artemisinin is greatly shortened, the yield is increased, and a solid and reliable drug supply guarantee is provided for large-scale malaria prevention and control.
These success stories are just the tip of the iceberg in the field of molecular manufacturing with whole-cell synthesis technology.
From the production of high value-added fine chemicals, to the preparation of environmentally friendly biofuels, to the creation of biological materials with special properties, whole cell synthesis technology with its unique advantages, is continuing to expand the boundaries of molecular manufacturing, creating more value for humanity.
Enzymatic Synthesis: Precision molecular “craftsman”
![Whole cell and enzymatic synthesis of innovative pathways 3 Chemoenzymatic synthesis of natural products: evolution of methods and strategies [J]. Chinese Journal of Synthetic Biology, 2024, 5(5): 913-940. (in Chinese)](https://www.gsh-world.com/blog/wp-content/uploads/2025/01/download-2-1.jpg)
Enzymatic synthesis, which is matched with whole-cell synthesis, is like a skilled “molecular craftsman”, displaying a unique “carving” technique in vitro.
Enzymes, as the core “craftsman” in this molecular synthesis feast, are a special type of protein with magical catalytic magic, which can drive chemical reactions with high precision under mild reaction conditions, and carefully carve the substrate molecules step by step into the target product.
Take synthetic breast milk oligosaccharides (HMOs) as an example, which are a class of sugar molecules with complex structures and important physiological functions that play an indispensable role in the healthy growth of infants.
Traditional chemical synthesis methods are often faced with many problems such as complicated steps, many by-products and poor stereoselectivity, which are difficult to meet the needs of large-scale industrial production.
Enzymatic synthesis stands out with its unique advantages. Scientists have carefully screened and optimized a series of enzymes with specific catalytic activity, such as glycoside hydrolase and glycosyltransferase, through in-depth research on the biosynthesis pathway of breast milk oligosaccharides.
These enzymes are like a trained “team of craftsmen” that can accurately identify and use specific sugar molecular substrates, and gradually build complex and diverse breast milk oligosaccharide molecules according to a preset “synthesis blueprint.”
The use of enzymatic synthesis technology can not only achieve the efficient preparation of breast milk oligosaccharides, but also ensure that the product has a high degree of purity and the correct three-dimensional structure, providing a strong technical support for the upgrading of infant formula milk powder, so that the milk powder is closer to the nutritional standards of breast milk, and help the healthy growth of babies.
Looking at the application in the pharmaceutical field, enzymatic synthesis has also shown great potential.
The chemical synthesis of many drug molecules, especially those with complex structures and chiral centers, is often challenging.
Taking the production of antibiotics as an example, the chemical structure of some antibiotic molecules contains multiple chiral centers, and the biological activity between the enantiomers may be very different, which requires a high degree of stereoselectivity in the synthesis process.
Enzymatic synthesis is just capable of this difficult task.
By using specific stereoselective enzymes, such as REDOX enzymes, transaminases, etc., the reaction process can be accurately controlled to generate only a single isomer with the required biological activity, avoiding the cumbersome chiral resolution steps in traditional chemical synthesis, and greatly improving the efficiency and purity of drug synthesis.
It opens up a new path for the development and production of new drugs.
These successful cases fully demonstrate the accuracy and efficiency of enzymatic synthesis in the field of molecular manufacturing.
Compared with whole cell synthesis, enzymatic synthesis is not limited by the complex metabolic network in the cell, can control the reaction process more accurately, avoid unnecessary side reactions, and has significant advantages for the synthesis of molecules with complex structures and high purity requirements.
At the same time, enzymatic synthesis reaction conditions are mild, usually at room temperature, normal pressure can be carried out, without high temperature, high pressure and other extreme conditions, not only reduce energy consumption, but also reduce the impact on the environment, in line with the current green chemistry development concept.
Successful case: The testimony of the theory landing
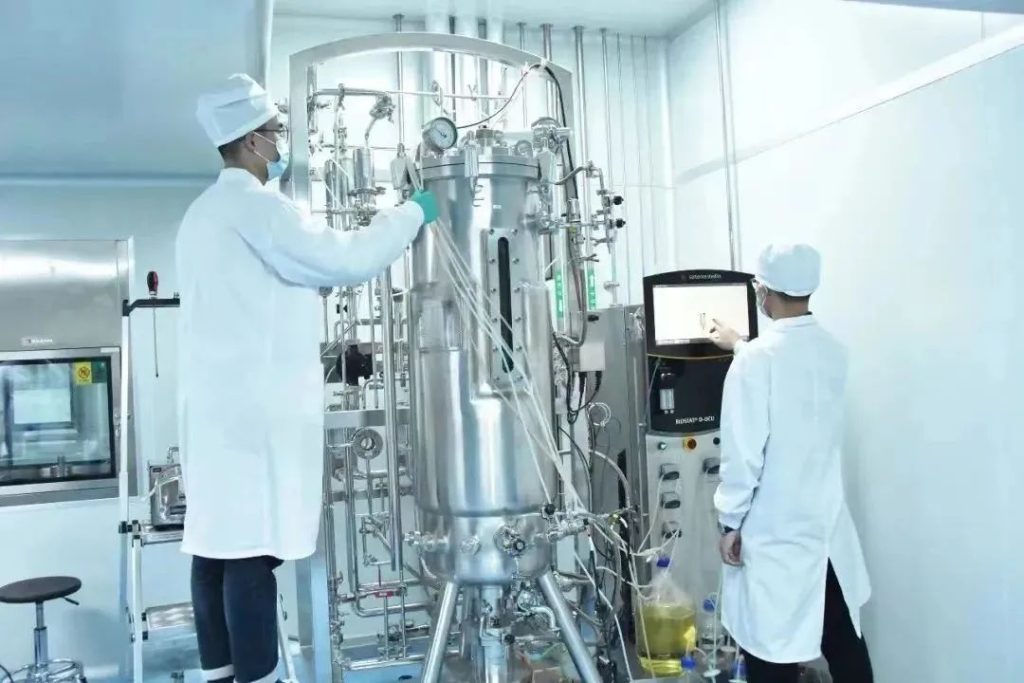
(1) Case analysis of whole cell synthesis
1. The creation of artificial cells
JCVI-syn3A, a synthetic cell created by a team from the J. Craig Venter Institute, the National Institute of Standards and Technology and the Massachusetts Institute of Technology, is a milestone in the quest for whole-cell synthesis.
Looking back, in 2010, the team took the lead in creating the first synthetic genome cell JCVI-syn1.0 in human history, a pioneering achievement that took more than 20 researchers nearly 15 years of hard work, during which the development of new technologies and new tools laid a solid foundation for subsequent research.
Since then, scientists have continued to work to simplify the genetic composition of cells, until 2016, the simplest cell JNCI-syn3.0 came out, its genome contains only 473 genes, but still can maintain basic life activities and self-replication.
However, JNCI-syn3.0 has defects in growth and division, and the daughter cells vary in shape and size.
In order to overcome this problem, the scientific research team started a five-year hard study, built dozens of variants by systematically adding and deleting genes, combined with a well-designed microfluidic thermostat to observe cell growth and division, and finally accurately identified 7 key genes and added them to JNCI-syn3.0. JCVI-syn3A was successfully bred.
This new type of cell can grow and divide normally like natural cells, which not only provides an excellent model for in-depth exploration of the mysteries of life, but also shows great potential in drug research and development, biological manufacturing and other fields, and is expected to become a “mini factory” for the production of drugs, food and fuel, and promote the revolutionary development of related industries.
2. Changes in starch synthesis
In China, the synthetic starch project completed by Ma Yanhe’s team at the Tianjin Institute of Industrial Biotechnology of the Chinese Academy of Sciences is also amazing.
For a long time, starch mainly relies on corn and other crops to fix carbon dioxide production through natural photosynthesis, the synthesis process involves more than 60 steps of complex metabolic reactions and fine physiological regulation, the theoretical energy conversion efficiency is only about 2%, and the crop planting cycle is long, requiring a lot of land, fresh water and agricultural production means.
In the face of these challenges, Ma Yanhe’s team since 2015, in conjunction with the Dalian Institute of Chemical Physics, Chinese Academy of Sciences, innovated a strategy similar to “building blocks” to design a new 11-step reaction of unnatural carbon dioxide fixing and synthetic starch pathway.
They first used chemical catalysts to reduce high concentration of carbon dioxide to a carbon compound under the help of high-density hydrogen energy, then carefully designed and constructed a new carbon-polymerization enzyme, polymerized into a carbon tricompound according to the principle of chemical glycan reaction, and then optimized and upgraded through biological pathways to gradually synthesize amylose and amylopectin.
The rate of synthetic starch synthesis in this way is as high as 8.5 times that of corn starch synthesis. According to the estimation of existing technical parameters, theoretically the annual starch output of 1 cubic meter bioreactor is equivalent to the starch output of 5 mu of corn cultivation land.
This achievement not only opens up a new road for starch production from traditional agricultural cultivation to industrial workshop manufacturing, but also Outlines a new blueprint for the synthesis of complex molecules of carbon dioxide raw materials, which has far-reaching impact on the future development of agriculture, food security and the global biofuturing industry, and is a well-deserved “from 0 to 1” original breakthrough.
(2) Enzymatic synthesis case selection
1. Enzymatic breakthrough of hyaluronic acid
In the field of enzymic synthesis, Israel’s company Enzymic production of hyaluronic acid is successfully successful, which has attracted wide attention from the enzymic industry.
As a kind of natural biopolymer widely found in skin, joint, connective tissue and nerve tissue, hyaluronic acid is widely used in many fields such as beauty care, ophthalmology, wound healing and rheumatism treatment.
In the past, the extraction of hyaluronic acid mainly relied on animal tissue extraction method, microbial fermentation method and chemical synthesis method, but these traditional methods have drawbacks, animal tissue extraction method of separation process is complicated, extraction rate is low, chemical synthesis of the product purity is not good, microbial fermentation method can set the molecular weight size on demand, but there are also complex processes, high cost problems.
Enzymit is adopting a new approach by designing and creating enzymes using advanced artificial intelligence technology.
Its proprietary enzyme design platform combines advanced computing and deep learning algorithms with precision experimental tools to create an efficient and economical solution tailored to cell-free enzyme manufacturing.
These new enzymes are resistant to high temperatures and can be recycled throughout the production cycle, greatly optimizing the production process.
Through enzymatic biological production, the company can accurately control the molecular weight range of hyaluronic acid, from low to high to meet the needs of different application scenarios, and cell-free manufacturing process significantly reduces the complexity, time cost and capital cost of traditional production methods, injecting a strong impetus for large-scale, high-quality production of hyaluronic acid, and promoting its wide application in more fields. To benefit human health and beauty.
2. Innovative synthesis of organic selenides
Professor Li Shengying’s team of Shandong University has made a major original breakthrough in the enzymatic synthesis of organic selenides, which has opened up a new world in the field of drug development.
Selenium and sulfur belong to the same sulfur element and have similar chemical properties. However, compared with organic sulfides, organic selenides have significant advantages in improving oxidation reduction, optimizing drug conformation, regulating drug metabolism, enhancing bioavailability and resisting drug resistance, etc.
In recent years, they have gradually become a popular direction in drug research and development.
However, natural organic selenides are scarce, and the chemical synthesis of chiral selenides is more difficult. Especially, the lack of effective means to construct “carbon-selenium bond” through enzyme catalysis has severely restricted the creation and development of organic selenides.
Professor Li Shengying’s team pioneered the “element engineering” strategy, based on the chemical principle of the natural sulfur carrier protein catalytic system, cleverly utilized the similarities and differences in the physical and chemical properties of selenium and sulfur, and successfully transformed the natural organic sulfide biosynthesis system into an organic selenide synthesis system.
The team carefully obtained 19 enzyme proteins related to cysteine, vitamin B1, and innovative mycin biosynthesis pathway through protein heterologous expression and purification, explored and verified whether “sulfur carrier protein” could be converted into “selenium carrier protein”, and finely optimized the reaction conditions.
Finally, the enzymatic synthesis of selenocysteine, selenovitamin B1 and selenochemycin derivatives was achieved for the first time through a multi-enzyme cascade catalytic reaction in vitro, providing a new and universal biocatalytic scheme for the creation of chiral organic selenides.
This breakthrough study not only fills the technical gap of organic selenide enzymatic synthesis, but also uses biocatalytic system to create more compounds containing rare life elements and even non-life elements, which is expected to give birth to more innovative drugs and escort human health.
Comparison of two: each has its own advantages of synthesis path
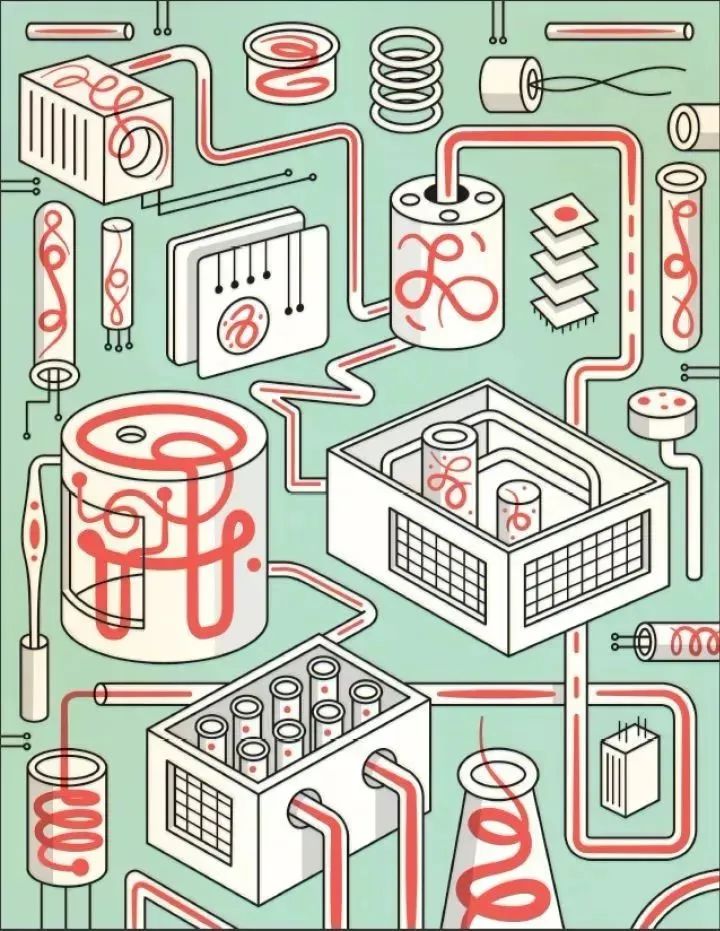
Both whole-cell synthesis and enzymatic synthesis are powerful “weapons” made of molecules, although they both glow and heat in the field of synthetic biology, but their modes of action are significantly different, just like two different styles of “craftsmen”, each good at different types of “molecular carving”.
From the perspective of synthetic environment, whole-cell synthesis relies on the complex and orderly physiological environment inside living cells, like a precise micro-factory, where numerous organelles, enzyme systems and metabolic pathways cooperate with each other to provide comprehensive support for the synthesis of target molecules.
However, this approach also means being limited by the cell’s own physiological characteristics, such as the cell’s growth cycle, metabolic regulation mechanisms, and sensitivity to environmental factors.
Once the environment of the cell changes, such as temperature, nutrient concentration, osmotic pressure, etc., deviates from the appropriate range, it may affect the normal growth and metabolism of the cell, and then interfere with the synthesis efficiency and quality of the target molecule.
Enzymatic synthesis, by contrast, is like a flexible “molecular workshop” built outside the body, free from the shackles of the cell’s overall physiological environment.
As the core catalyst, enzymes can precisely drive chemical reactions under relatively simple and easily controlled conditions such as the appropriate buffer system, temperature, and pH value.
This allows enzymatic synthesis to be more flexible and fine-tune the reaction conditions according to the needs of the target molecule, avoiding the uncertainty caused by complex factors in the cell.
In terms of reaction accuracy and substrate selectivity, enzymatic synthesis shows a high accuracy by virtue of the unique active center structure of enzyme molecules. Like a “sniper” with bright eyes, it can accurately identify and combine substrate molecules with specific structures, catalyze specific chemical reactions, strictly follow the preset reaction path, and rarely produce by-products. The high purity of the product and the precise construction of the specific structure are ensured.
For example, in the synthesis of certain drug molecules with chiral centers, the enzyme can generate only a single isomer with biological activity by its high stereoselectivity, avoiding the cumbersome and inefficient chiral resolution step in traditional chemical synthesis.
As for whole-cell synthesis, due to the complexity of intracellular metabolic network, it is like a huge and busy “comprehensive processing plant”, where numerous metabolic pathways are intertwined, and substrates may participate in various metabolic reactions in the cell. Although the cell itself has certain regulatory mechanisms, its reaction accuracy and substrate selectivity are relatively low compared with enzymatic synthesis. More likely to produce some unwanted by-products.
However, it is the complexity of this metabolic network that also gives whole cell synthesis a certain advantage, enabling it to use relatively simple and cheap substrates, through a series of metabolic transformations, to synthesize more complex structure of the target molecule.
Cost-effectiveness is also an important index to measure the two synthesis methods.
Whole cell synthesis often presents significant cost advantages in the mass production of relatively simple molecules that are in high demand.
Taking the common industrial fermentation production of amino acids as an example, the use of genetically engineered microbial cells can use low-cost sugars and nitrogen sources as raw materials, through large-scale fermenter culture, to achieve efficient production of amino acids.
This is because cells can use their own metabolic system to continuously convert raw materials into target products, and with the expansion of production scale, the cost per unit of product can be effectively shared.
However, for some molecules with high added value, complex structure and high purity requirements, whole cell synthesis may require more costs for the optimization of cell culture conditions and the separation and purification of impurities.
At this time, enzymatic synthesis may be more economical, although the preparation and screening of enzymes itself requires a certain cost input, but due to its high catalytic efficiency, accurate reaction control and relatively simple post-treatment process, it can reduce raw material waste and energy consumption when synthesizing high-purity, high-value-added products, thus reducing the total cost in long-term operation.
In summary, whole cell synthesis and enzymatic synthesis are not substitutes for each other, but complement each other.
In the actual molecular research and development and production process, researchers need to carefully weigh the characteristics of the target molecules, production scale, cost budget and requirements for product purity and structure, and other factors, cleverly choose or combine the two synthesis strategies, in order to give full play to their advantages and achieve the optimal solution of molecular manufacturing.
Promote the wide application and in-depth development of synthetic biology in various fields.
Future Vision: The frontier of infinite possibilities
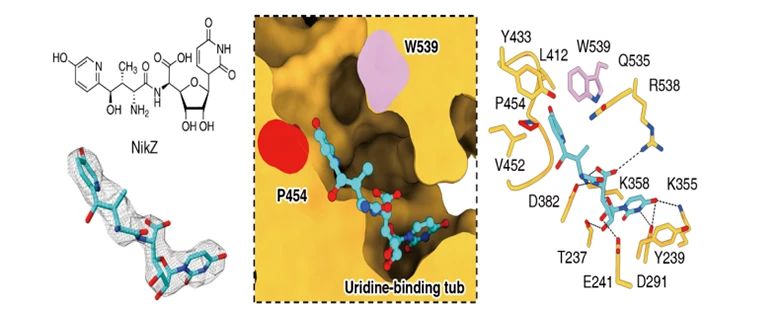
Looking forward to the future, the prospect of synthetic biology in the field of molecular research and development is like a vast star, full of infinite possibilities.
With the continuous breakthrough and innovation of technology, the two “swords” of whole cell synthesis and enzymatic synthesis will be sharper and create more miracles for human beings.
In the field of medicine, we have reason to expect more personalized and precise drug treatment programs to become a reality.
Using synthetic biology, scientists are able to tailor drugs that work best with the least side effects based on a patient’s unique genetic information.
Imagine that the future of cancer treatment is no longer a “one-size-fits-all” traditional model, but through the in-depth analysis of cancer cells, designed specifically for the specific target of cancer cells, “smart drugs”, these drugs can be like precision guided missiles, directly hit the vital point of cancer cells, while maximizing the protection of normal cells from damage. For patients to bring more survival hope and higher quality of life.
Chemicals are also poised for a green revolution. Bio-based materials will gradually replace traditional petroleum-based materials and become the mainstream.
Green products such as degradable plastics and biofuels will be widely used in all aspects of daily life, effectively alleviating environmental pollution problems, and helping mankind move towards a better future of sustainable development.
Perhaps in the near future, the plastic products in our hands will be derived from renewable biological resources, which can be rapidly degraded naturally after use, and no longer leave the earth with “white garbage” that is difficult to eliminate;
Cars will also be fueled more by efficient and clean bioenergy, significantly reducing carbon emissions and making blue skies and white clouds the norm.
The food field will also take on a new look, artificial meat, artificial milk and other new foods will be closer to the quality and taste of natural foods, to meet the growing nutritional needs of people, while reducing the pressure of traditional animal husbandry on the environment.
We can foresee that in the future, the artificial meat burger not only looks and tastes the same as the traditional beef burger, but also contains more high-quality protein and nutrients, and its production process does not need to occupy a lot of land, water resources, nor produce too much greenhouse gas emissions, truly realize the perfect integration of delicious and environmental protection.
Synthetic biology still faces many challenges on the road of molecular research and development, such as technical bottlenecks, ethical considerations, and regulatory challenges.
But like every technological change in history, difficulties only inspire human wisdom and courage, and push us forward.
About GSHWORLD